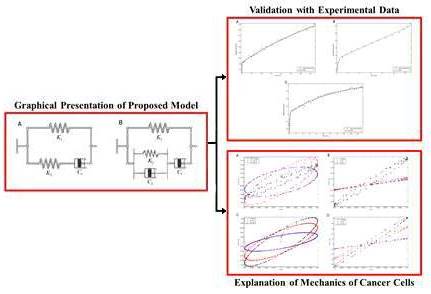
Specific mechanical behaviours of healthy and cancer cells via a new model for cells and actin networks
Abstract
Understanding the viscoelastic nature of the cells and their response to the mechanical stimuli provides the knowledge to analyse some behaviours of the cells. Although different elements of the cell are important for its mechanical properties but experimental data highlights actin cytoskeleton as the most important element. In this study, experimental data from previous studies including storage and loss moduli of different actin networks, networks with different cross-linkers or without them, and also creep behaviour of different cells are extracted to propose a new model. This newly presented model provides an insight to the viscoelastic properties of both actin networks and cells which are closely related to each other. It can be pointed out that the ability of this model to define the relations between concentration of actin or its cross-linkers and the adjustable parameters of the model, makes it a generic model. This model could be used to explain different mechanical behaviours of the healthy and cancer cells, including the decrease in the stiffness of the cancer cells, in terms of the changes in cross-linkers’ concentration. From biomechanical view, changes in actin cross-linkers’ concentration or type is one of the most important variations in cancer cells during their malignant transformation, which greatly affects their behaviours such as extracellular matrix detachment, deformability, and mobility. The knowledge of these dis-regulations of cancer tissue could be useful in their prediction, diagnosis, and treatment.
Full Text:
PDFReferences
Ethier, C.R. and C.A. Simmons, Introductory Biomechanics: From Cells to Organisms. 2007: Cambridge University Press.
Alberts, B., Molecular Biology of the Cell. illustrated ed. 2008: Garland Science.
MacKintosh, F.C., J. Kas, and P.A. Janmey, Elasticity of semiflexible biopolymer networks. Phys Rev Lett, 1995. 75(24): p. 4425-4428.
Xu, J., Y. Tseng, and D. Wirtz, Strain hardening of actin filament networks. Regulation by the dynamic cross-linking protein alpha-actinin. J Biol Chem, 2000. 275(46): p. 35886-92.
Sato, M., et al., Application of the micropipette technique to the measurement of cultured porcine aortic endothelial cell viscoelastic properties. J Biomech Eng, 1990. 112(3): p. 263-8.
Gardel, M.L., et al., Prestressed F-actin networks cross-linked by hinged filamins replicate mechanical properties of cells. Proc Natl Acad Sci U S A, 2006. 103(6): p. 1762-7.
Ward, I.M. and J. Sweeney, An Introduction to the Mechanical Properties of Solid Polymers. 2 ed. 2005: Wiley.
Pardee, J.D. and J.A. Spudich, Chapter 18 Purification of Muscle Actin, in Methods in Cell Biology, W. Leslie, Editor. 1982, Academic Press. p. 271-289.
Schafer, D.A., P.B. Jennings, and J.A. Cooper, Rapid and efficient purification of actin from nonmuscle sources. Cell Motil Cytoskeleton, 1998. 39(2): p. 166-71.
Lieleg, O., M.M. Claessens, and A.R. Bausch, Structure and dynamics of cross-linked actin networks. Soft Matter, 2010. 6(2): p. 218-225.
Walcott, S. and S.X. Sun, A mechanical model of actin stress fiber formation and substrate elasticity sensing in adherent cells. Proc Natl Acad Sci U S A, 2010. 107(17): p. 7757-62.
Lieleg, O., et al., Transient binding and dissipation in cross-linked actin networks. Phys Rev Lett, 2008. 101(10): p. 108101.
Deng, L., et al., Fast and slow dynamics of the cytoskeleton. Nat Mater, 2006. 5(8): p. 636-40.
Fabry, B., et al., Time scale and other invariants of integrative mechanical behavior in living cells. Phys Rev E Stat Nonlin Soft Matter Phys, 2003. 68(4 Pt 1): p. 041914.
Cai, P., et al., Quantifying cell-to-cell variation in power-law rheology. Biophys J, 2013. 105(5): p. 1093-102.
Schmid-Schönbein, G.W., et al., Passive mechanical properties of human leukocytes. Biophys J, 1981. 36(1): p. 243-256.
Elson, E.L., Cellular mechanics as an indicator of cytoskeletal structure and function. Annu Rev Biophys Biophys Chem, 1988. 17: p. 397-430.
Pachenari, M., et al., Mechanical properties of cancer cytoskeleton depend on actin filaments to microtubules content: investigating different grades of colon cancer cell lines. J Biomech, 2014. 47(2): p. 373-9.
Drury, J.L. and M. Dembo, Aspiration of human neutrophils: effects of shear thinning and cortical dissipation. Biophys J, 2001. 81(6): p. 3166-77.
Liao, D., et al., Viscoelastic properties of isolated rat colon smooth muscle cells. Cell Biol Int, 2006. 30(10): p. 854-8.
Pravincumar, P., D.L. Bader, and M.M. Knight, Viscoelastic cell mechanics and actin remodelling are dependent on the rate of applied pressure. PLoS One, 2012. 7(9): p. e43938.
Khatibzadeh, N., et al., Effects of plasma membrane cholesterol level and cytoskeleton F-actin on cell protrusion mechanics. PLoS One, 2013. 8(2): p. e57147.
Cortés, F. and M.J. Elejabarrieta, Modelling viscoelastic materials whose storage modulus is constant with frequency. INT J SOLIDS STRUCT, 2006. 43(25): p. 7721-7726.
Schmidt, F.G., B. Hinner, and E. Sackmann, Microrheometry underestimates the values of the viscoelastic moduli in measurements on F-actin solutions compared to macrorheometry. Phys Rev E Stat Phys Plasmas Fluids Relat Interdiscip Topics, 2000. 61(5 Pt B): p. 5646-53.
Kasza, K.E., et al., Actin filament length tunes elasticity of flexibly cross-linked actin networks. Biophys J, 2010. 99(4): p. 1091-100.
Luan, Y., et al., Micro- and macrorheological properties of isotropically cross-linked actin networks. Biophys J, 2008. 94(2): p. 688-93.
Ward, S.M., et al., Dynamic viscoelasticity of actin cross-linked with wild-type and disease-causing mutant alpha-actinin-4. Biophys J, 2008. 95(10): p. 4915-23.
Janmey, P.A., et al., The mechanical properties of actin gels. Elastic modulus and filament motions. J Biol Chem, 1994. 269(51): p. 32503-13.
Schmoller, K.M., O. Lieleg, and A.R. Bausch, Structural and viscoelastic properties of actin/filamin networks: cross-linked versus bundled networks. Biophys J, 2009. 97(1): p. 83-9.
Xu, J., A. Palmer, and D. Wirtz, Rheology and microrheology of semiflexible polymer solutions: actin filament networks. Macromolecules, 1998. 31(19): p. 6486-6492.
Stevenson, R.P., D. Veltman, and L.M. Machesky, Actin-bundling proteins in cancer progression at a glance. J Cell Sci, 2012. 125(Pt 5): p. 1073-9.
Zamir, E. and B. Geiger, Components of cell-matrix adhesions. J Cell Sci, 2001. 114(Pt 20): p. 3577-9.
Gabbiani, G., et al., Contractile proteins in human cancer cells. Immunofluorescent and electron microscopic study. Am J Pathol, 1976. 83(3): p. 457-74.
Jiang, X., et al., Inhibition of filamin-A reduces cancer metastatic potential. Int J Biol Sci, 2013. 9(1): p. 67-77.
Yue, J., S. Huhn, and Z. Shen, Complex roles of filamin-A mediated cytoskeleton network in cancer progression. Cell Biosci, 2013. 3(1): p. 7.
Yamamoto, S., et al., Actinin-4 gene amplification in ovarian cancer: a candidate oncogene associated with poor patient prognosis and tumor chemoresistance. Mod Pathol, 2009. 22(4): p. 499-507.