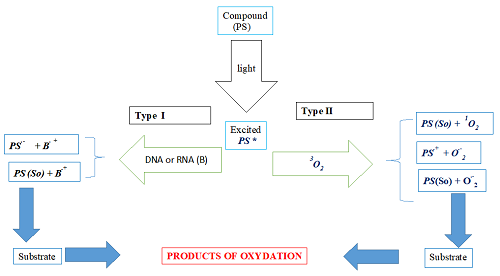
Theoretical study of two coumarin derivatives photosensitivity for possible use in photodynamic therapy
Abstract
The dynamism of cancer and its side effects related to different treatments are real questions for humankind to solve. Thus, this manuscript aims to explore the photochemical and photo-physical properties of two coumarin molecules due to their multiple biological and spectroscopic activities 1 in the framework of photodynamic therapy (PDT) as a photosensitizer (PS). For our aim fulfillment, quantum chemical methods such as DFT and TD-DFT at the B3LYP/6-31G(d,p) level were used in different media 2 to determine the parameters quoted above. The obtained results show that the solvent's nature influences the compounds' photosensitivity 3. Thus, both compounds M1 and M2 are coumarins. M1 and M2 belong to benzocoumarin and simple coumarin families, respectively. In other words, the coumarin ring of M1 is attached to a benzene ring. Apart from this difference, compound M1 contains a triazol ring, and compound M2 contains an oxadiazol ring. These compounds produce charged radicals.
Moreover, compound M1 presents the lowest values of VIP and the energy of the excited state ET necessary for producing charged radicals. Therefore, it is assumed to be the most photosensitive, and this photosensitivity is more accentuated in polar solvents. In sum, studied coumarins, in addition to being used in chemotherapy, can also be used in PDT as PS. However, the theoretical improvement of the studied parameters would be a significant advance for the experimenter.
Full Text:
PDFReferences
L. Ouattara, K. Bamba, M.G.R. Koné, J.S. N’dri, K.N. N’Guessan, O.P. Massapihanhoro, D. Fatogoman, Predictive Modeling of Breast Anticancer Activity of a Series of Coumarin Derivatives using Quantum Descriptors, Chem. Sci. Int. J., 2019, 26, 1–10.
E.K. Akkol, Y. Genç, B. Karpuz, E. Sobarzo-Sánchez, R. Capasso,Coumarins and coumarin-related compounds in pharmacotherapy of cancer, Cancers, 2020, 12, 1–25.
A.F. dos Santos, D.Q. de Almeida, L.F. Terra, M.S. Baptista, L. Labriola, Photodynamic therapy in cancer treatment - an update review,
J. Cancer Metastasis Treat., 2019, 25, 1–20.
P. Wyss, V. Schwarz, D. Dobler‐Girdziunaite, R. Hornung, H. Walt, A. Degen, M. Fehr, photodynamic therapy of locoregional breast cancer recurrences using a chlorin-type photosensitizer, Int. J. Cancer, 2001, 93,
–724.
T. Dudzik, I, Domanski, S. Makuch, The impact of photodynamic therapy on immune system in cancer – an update, Front. Immunol., 2024, 15, 1335920.
Q. Chen, L. Xu, C. Liang, C. Wang, R. Peng, Z. Liu, Photothermal therapy with immune-adjuvant nanoparticles together with checkpoint blockade for effective cancer immunotherapy, Nat Commun., 2016, 7, 13193.
B. Farhood, M. Najafi, K. Mortezaee, CD8+ cytotoxic T lymphocytes in cancer immunotherapy: A review, J Cell Physiol., 2019, 234, 8509- 8521.
S.O. Gollnick, L. Vaughan, B.W. Henderson, Generation of effective antitumor vaccines using photodynamic therapy, Cancer Res., 2002, 62, 1604-1608.
A.K. D'Cruz, M.H. Robinson, M.A. Biel, mTHPC-mediated photodynamic therapy in patients with advanced, incurable head and neck cancer: A multicenter study of 128 patients, Head Neck, 2004, 26, 232-240.
S.A. Morsy, A.A. Farahat, M.N.A. Nasr, A.S. Tantawy, Synthesis, molecular modeling and anticancer activity of new coumarin containing compounds, Saudi Pharm. J., 2017, 25, 873–883.
S. Kwiatkowski, B. Knap, D. Przystupski, J. Saczko, E. Kędzierska, K., Knap-Czop, J. Kulbacka, Photodynamic therapy – mechanisms, photosensitizers and combinations, Biomed. Pharmacother., 2018, 106, 1098–1107.
R.R. Allison, K. Moghissi, Photodynamic therapy (PDT): PDT mechanisms, Clin. Endosc., 2013, 46, 24–29.
C.A. Robertson, D.H. Evans, H. Abrahamse, Photodynamic therapy (PDT): A short review on cellular mechanisms and cancer research applications for PDT, J. Photochem. Photobiol. B Biol., 2009, 96, 1–8.
A.P. Castano, T.N. Demidova, M.R. Hamblin, Mechanisms in photodynamic therapy: Part two - Cellular signaling, cell metabolism and modes of cell death, Photodiagnosis Photodyn. Ther., 2005, 2, 1–23.
L. Shen, H.F. Ji, H.Y. Zhang, A TD-DFT study on triplet excited-state properties of curcumin and its implications in elucidating the photosensitizing mechanisms of the pigment, Chem. Phys. Lett., 2005, 409, 300–303.
M. Cossi, V. Barone, R. Cammi, J. Tomasi, Ab initio study of solvated molecules: A new implementation of the polarizable continuum model, Chem. Phys. Lett., 1996, 255, 327–335.
Y. Valadbeigi, H. Farrokhpour, M. Tabrizchi, G4MP2, DFT and CBS-Q calculation of proton and electron affinities, gas phase basicities and ionization energies of hydroxylamines and alkanolamines, J. Chem. Sci., 2014, 4, 1209–1215.
X. Zhao, Z. Zheng, S. Feng, Z. Shi, D. Chen, A TD-DFT study on the photo-physicochemical properties of chrysophanol from rheum, Int.
J. Mol. Sci., 2009, 10, 3186–3193.
C.M.N. Yow, N.K. Mak, S. Szeto, J.Y. Chen, L.Y. Lee, N.H. Cheung, A.W.N. Leung, Photocytotoxic and DNA damaging effect of Temoporfin ( mTHPC ) and merocyanine 540 (MC540 ) on nasopharyngeal carcinoma cell, Toxicol. Lett., 2000, 115, 53–61.
W.P. Ouattara, K. Bamba, A.S. Thomas, F. Diarrassouba, L. Ouattara, M.P.Ouattara, N. Zia, Theoretical Studies of Photodynamic Therapy Properties of Azopyridine δ-OsCl2(Azpy)2 Complex as a Photosensitizer by a TDDFT Method Wawohinlin, Comput. Chem., 2021, 9, 64–84.
E. Ortega-Forte, A. Rovira, A. Gandioso, J. Bonelli, M. Bosch, J. Ruiz, V. Marchán, COUPY Coumarins as Novel Mitochondria-Targeted Photodynamic Therapy Anticancer Agents, J. Med. Chem., 2021, 64, 17209−17220.
L. Shen, H.F. Ji, H.Y. Zhang, A TD-DFT study on photo-physicochemical properties of hypocrellin A and its implications for elucidating the photosensitizing mechanisms of the pigment, J. Photochem. Photobiol. A Chem., 2006, 180, 65–68.
B. Kafoumba, O.W. Patrice, D. Fatogoma, L. Ouattara, M.P. Ouattara, K.N. N'guessan, E.A. Donatien, Z. Nahossé, Theoretical Determination of Influence of the Metallic State of Oxidation toward Cytotoxic Activity: Case of Ruthenium Complexes. Computational Chemistry, 2021, 9, 97-119.
R.C. Evans, P. Douglas, H.D. Burrows, Applied photochemistry, Dordrecht: Springer, 2014, 36.
S. Perun, J. Tatchen, C.M. Marian, Singlet and triplet excited states and intersystem crossing in free-base porphyrin: TDDFT and DFT/MRCI study, ChemPhysChem, 2008, 9, 282–292.
J. Llano, J. Raber, L.A. Eriksson, Theoretical study of phototoxic reactions of psoralens, J. Photochem. Photobiol. A Chem., 2003, 154, 235–243.
W. Wu, X. Shao, J. Zhao, M. Wu, Controllable Photodynamic Therapy Implemented by Regulating Singlet Oxygen Efficiency, Adv. Sci., 2017, 4, 1–21.
B. Halliwell, Antioxidant defense mechanisms: From the beginning to the end (of the beginning), Free Radic. Res., 1999, 31, 261–272.
DOI: http://dx.doi.org/10.13171/mjc02408061795ouattara
Refbacks
- There are currently no refbacks.
Copyright (c) 2024 Mediterranean Journal of Chemistry